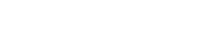
New Evidence: Earth Had Rings (and Might Regain Them)
Season 11 Episode 1 | 16m 30sVideo has Closed Captions
There’s an extremely good chance that Earth once did have a ring system.
There’s an extremely good chance that Earth once did have a ring system. At least, that’s the proposal by a recent study that has evidence that a mysterious burst in meteor activity nearly half a billion years ago was actually caused by that ancient ring system collapsing onto the Earth. And, you know, if we had a ring once maybe we can have one again.
Problems with Closed Captions? Closed Captioning Feedback
Problems with Closed Captions? Closed Captioning Feedback
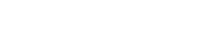
New Evidence: Earth Had Rings (and Might Regain Them)
Season 11 Episode 1 | 16m 30sVideo has Closed Captions
There’s an extremely good chance that Earth once did have a ring system. At least, that’s the proposal by a recent study that has evidence that a mysterious burst in meteor activity nearly half a billion years ago was actually caused by that ancient ring system collapsing onto the Earth. And, you know, if we had a ring once maybe we can have one again.
Problems with Closed Captions? Closed Captioning Feedback
How to Watch PBS Space Time
PBS Space Time is available to stream on pbs.org and the free PBS App, available on iPhone, Apple TV, Android TV, Android smartphones, Amazon Fire TV, Amazon Fire Tablet, Roku, Samsung Smart TV, and Vizio.
Providing Support for PBS.org
Learn Moreabout PBS online sponsorshipPlanet Earth is the jewel of the solar system—the shimmery blue oceans, the verdant green forests, the wispy whimsical cloud formations.
Saturn would be the only competitor for most gorgeous planet with that giant ring system.
But you know what?
If we could put the jewel of the Earth in its own ring?
There'd be no contest.
Well, there’s an extremely good chance that Earth once did have a ring system.
And, you know, if we had a ring once maybe we can have one again.
Ever since they were discovered by Galileo and then seen for their true majesty by Christiaan Huygens, Saturn’s rings have been a symbol of serene celestial order.
They seem so timeless and eternal.
But they are more transient than they look.
Saturn will lose its own ring system in a few hundred million years, and a hundred million years or so ago an astronomer with a time machine, or I guess a caveman with a telescope would probably have seen Saturn as a ringless yellow ball.
Jupiter, Uranus and Neptune all have vestigial rings—perhaps the remnants of greater systems.
There’s even a hypothesis that Mars goes through cycles of having rings versus moons.
So, if planetary rings come and go, it stands to reason that Earth may have once had one.
And now we have evidence that it really did, at least according to a 2024 study by scientists from Monash University, in my own home town actually, Melbourne, Australia.
But before we get to that evidence, let’s learn a bit about how planetary rings can form and how they can go away.
We’ll start by imagining a hypothetical planet that already has a ring system.
Those rings are just collections of particles; rocks, ice, or dust with circular orbits in a flat plane.
There are a couple of ways this ring could be lost.
One is that the particles fall onto the planet due to various factors, including gravitational, magnetic and even atmospheric interactions.
That’s actually inevitable, but can take a really long time.
A quicker way to remove a ring is to turn it into moons.
Even though the particles of a ring system particles are small, they attract each gravitationally and can slowly clump together into massive bodies.
Because the force of gravity is so weak between these particles, this can only happen if they’re moving very slowly relative to each other.
Larger relative speeds they’ll just wizz past each other before gravity can do its thing.
We say that a system of particles with large and random relative speeds is dynamically hot, otherwise it’s dynamically cold.
But even if a ring system starts out hot, collisions between particles can slow relative speeds to the point where they do start to coalesce gravitationally.
At that point they can build moons.
But if collisions can’t reduce particles speeds then the system remains hot and also remains a ring.
But even if a ring system cools, there’s still a way for it to stay a ring.
For example, Saturn’s rings are dynamically cold in fact, they may have the minimum possible internal energy that they could possibly have.
The other thing that can keep a ring a ring is the gravitational field of the planet itself.
Let’s Imagine some particles just starting to clump together into a ring system, with the grand ambition of forming a new moon.
As the clump grows, the mutual gravitational field holding it together gets stronger.
But the planet also has a gravitational field, and that field is stronger the closer you get to the planet.
That means particles in our growing clump on the side nearer to the planet feel a stronger inward pull than those on the far side.
As long as we place this body far from the planet, that gravity differential is be weaker than the gravity force holding the clump together.
But the closer to the planet we place it, the stronger this differential becomes.
Within a certain range, the difference in the force of gravity across the body’s width is stronger than the force of gravity holding the body together.
That causes the growing clump to breaks apart—or to not grow in the first place.
This differential force is called a tidal force and our Moon’s differential pull on Earth’s oceans is the cause of tides.
So we can have rings if the material is dynamically hot OR if the material is inside this critical distance for tidal disruption.
That distance is called the Roche limit, and it depends on a few factors, like the density of material forming the moon and whether the material is held together by anything other than gravity.
Within the Roche limit for a given planet and a given material, Moons aren’t possible and so orbiting particles will persist as rings.
Beyond the Roche limits, these particles will eventually form moons as long as they can also become dynamically cool.
For Saturn, the Roche limit for ice is around 140 thousand kilometres from the planet’s centre.
There are no moons inside this boundary, and there is only very thin ring material outside of it.
There are two main ways for a planet to gain rings.
One is to capture small particles that arrive elsewhere in the solar system, which then fail to coalesce into moons, for example due to tidal forces.
Or a larger object could be shattered into smaller particles while orbiting or on a near fly-by—again perhaps by tidal forces or through a collision.
There are other possible scenarios, like a giant impact with the planet itself, spraying particles from the planet into a ring system.
Supposedly that’s how Earth’s moon was formed, although in this case the “ring system” didn’t last long because it was outside Earth’s Roche limit.
But in principle, Earth could acquire a persistent ring system as long as it was within its Roche limit.
So let’s look at the new evidence that this was, in fact, once the case.
We’ve known for some time that around 4 and half Million years ago a bunch of stuff fell to Earth.
Space rocks are peppering our planet constantly, and we see their remains—both in impact craters, but also in more broadly dispersed meteorite material in sedimentary rock, such as limestone.
In fact, from these rocks we can trace the flux of meteorites—the rate of infall.
And for a period of around 40 million years starting around 466 million years ago, sediment became enriched with a type of space rock called L-chondrite.
Enriched by a factor of 100-1000.
So, for this stretch of some tens of millions of years during the Ordovician period, the sky blazed with shooting stars … for some reason.
This is the Ordovician impact spike Perhaps the most obvious explanation is that there was some massive influx of material into the inner solar system from the asteroid belt.
You expect that sort of thing when two large asteroids collide.
In fact, that was, and perhaps, for the time being, still is the leading hypothesis for the excess L-chondrite deposits.
Except there’s something that doesn’t quite hang together.
If this event really did occur in the asteroid belt, we’d expect all inner solar system bodies to be affected.
But there’s no evidence of an increased impact rate on Mars or the Moon from this period.
This incongruity is part of what led the researchers from Monash university to look into this idea that the many, many smallish meteorites that mark the Ordovician impact spike were produced close to the Earth instead of in the asteroid belt.
So here’s their story: about 466 million years ago, a large asteroid – about 10 kilometres across – had a near miss with the Earth.
It passed just thousands of kilometers above the planet’s surface, below the Roche limit, where extreme tidal forces disrupted the body.
Some of the resulting cloud of debris was then captured by the Earth’s gravity.
At first, these particles would have slung around the Earth on very elliptical orbits and with a range of inclinations.
At first, the scattered orbital orientations led to frequent collisions, but those collisions circularized the orbits and helped flattened them into a disk.
Earth’s slight equatorial bulge also creates a preferential orbital plane around the equator, further stabilizing orbits into a very flat ring system.
Now some particles ended up outside the Roche limit may have temporarily formed small moons out there.
But tidal forces would have caused them to migrate inwards until they were once again tidally disrupted and rejoined the inner ring.
But that ring could not last forever.
Earth’s Roche limit is a few thousand kilometers for solid rock asteroid and up to 15 or so thousand clicks for a fragmented asteroid—a “rubble pile”.
Either way, an object that breaks up with this range is also largely inside Earth’s exosphere.
That’s the extremely diffuse region where the atmosphere transitions into interplanetary space.
The tiny drag caused by interactions with the exosphere would have caused these ring orbits to slowly decay.
One by one, ring particles would find themselves in an accelerating spiral into the thickening atmosphere, ending in a fiery plummet to the surface.
So, if this story is right, then for about 40 million years the Earth had a spectacular ring stretching thousands of kilometers above the equator, and also a constant rain of shooting stars from the base of that slowly draining ring.
Well cool story.
But how do we test something like this?
How do we tell that this stuff that fell from space came from a ring rather than from a collision in the asteroid belt, given that it would all be the same type of material?
Well the trick is to see where the stuff landed.
Material coming direction from the asteroid belt should land relatively randomly over the entire surface of the globe.
On the other hand, material falling from a ring should land close to the equator.
It’s not exactly easy to say where space rocks landed half a billion years ago.
The tiny bits of L-chondrite found in sedimentary layers have dispersed widely from their atmospheric entry points.
But there are several actual craters thought to be caused by larger impacts from this event.
And these are scattered across the globe, from Europe to North America to Australia, so definitely not equatorial.
But remember that these craters formed a half billion years ago, when the continents were nowhere near their current locations.
So the researchers traced the tectonic motion of these impact craters back in time to see where they were located when they were formed.
And yes, it’s crazy that we can do that.
Back then, most of Earth’s solid surface was clustered in the southern hemisphere supercontinent Gondwana.
And the impact locations?
All of them within roughly 30 degrees latitude of the equator.
Just what you’d expect from a collapsed planetary ring.
But let’s not get ahead of ourselves.
It’s only 21 craters, and the range is around 60 degrees latitude.
Maybe there really was an influx of rocks from the asteroid belt, and all the bigger ones just got lucky and landed in the tropics by accident.
What are the chances?
Well the team calculate the chances.
The likelihood of getting all of these impacts within this band around the equator is reported as one in 25 million.
If they really were from a global random distribution that is.
Therefore, these researchers argue that it’s much more likely that these impacts came from a reservoir of space rocks localised above the equator of the Earth.
AKA a planetary ring.
It seems … plausible.
Not super easy to test, unfortunately and I'll come back to how we might test this hypothesis.
But it does feel reasonable.
And the idea of a planetary ring does at least seem consistent with the general state of affairs 2.6 hundred million years ago.
So then animal life was all marine at that time during the Ordovician period, was busily complexifying in preparation for its foray onto land.
And that land was clustered in the Gondwana supercontinent, which spent most of this era covered in primitive plantlife.
But towards the end became a colossal ice-cap as Earth plunged into the Hirnantian glaciation.
This ice age was so bad that it led to the second largest mass extinction in Earth’s history.
The Ordovician impact spike came just before the Hirnantian glaciation, and others have proposed that the asteroid influx precipitated the cooling by depositing a lot of reflective dust in the atmosphere.
But the planetary ring hypothesis may provide an even more compelling explanation for the cooling.
That’s because an equatorial ring would provide a sunshade for whichever hemisphere happened to be in winter.
That would result in harsher winters, and potentially initiate the runaway growth of Gondwana glaciers that precipitated the ice age .
And by the way, if you want a deeper look into what would happen to the modern Earth if it had rings, check out Joe Scott’s episode where he explores exactly that.
And in the process produced amazing visualizations of what a ringed Earth would look like, some of which we borrowed for this episode.
So did the ancient Earth have a ring system?
We can’t say one way or the other just yet.
The authors suggest various ways to strengthen the hypothesis or refute it.
The most obvious thing is to find more impact craters from this era, and refine our dating of the meteorite material in those craters, and of the mineral similarity of this material.
But if we found craters of the same type of meteorite and time very far from the equator it would put this hypothesis in doubt, but if we keep finding sibling craters that were close to the Ordovician equator then things look more promising.
And if it does turn out that Earth had a ring system a half billion years ago, then there’s no reason we can’t have one again.
All we need is a horrifyingly near-miss with an asteroid followed by millions of years of intense meteor activity and a cataclysmic ice age.
But it's a small price to pay to firmly establish Earth as the most spectacular world in local space time.
Support for PBS provided by: