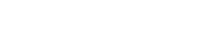
The Missing Mass Mystery
Season 3 Episode 45 | 11m 2sVideo has Closed Captions
Astronomers haven't been able to find up to half of the baryonic matter in the universe.
For years, astronomers have been unable to find up to half of the baryonic matter in the universe. We may just have solved this problem.
Problems with Closed Captions? Closed Captioning Feedback
Problems with Closed Captions? Closed Captioning Feedback
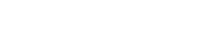
The Missing Mass Mystery
Season 3 Episode 45 | 11m 2sVideo has Closed Captions
For years, astronomers have been unable to find up to half of the baryonic matter in the universe. We may just have solved this problem.
Problems with Closed Captions? Closed Captioning Feedback
How to Watch PBS Space Time
PBS Space Time is available to stream on pbs.org and the free PBS App, available on iPhone, Apple TV, Android TV, Android smartphones, Amazon Fire TV, Amazon Fire Tablet, Roku, Samsung Smart TV, and Vizio.
Providing Support for PBS.org
Learn Moreabout PBS online sponsorship[MUSIC PLAYING] For years, astronomers have been unable to find up to half of the matter in the universe.
The missing baryon problem put into question understanding of the physics of the Big Bang.
We may just have solved it.
Fingers crossed.
[MUSIC PLAYING] Our astronomical surveys have revealed an observable universe full of hundreds of billions of galaxies, each of them with as many stars.
The shining light of these stars illuminates or is conspicuously absorbed by gas and dust within those galaxies.
When we extrapolate observations to the entire observable When we extrapolate observations to the entire observable universe, we find a billion trillion suns worth of mass.
universe, we find a billion trillion suns worth of mass.
universe, we find a billion trillion suns worth of mass.
However, we've known for some time that around 95% However, we've known for some time that around 95% of the energy content of the universe is in dark matter and dark energy.
This dark sector doesn't interact with light in any way and so is invisible to us.
The remaining 5%, the light sector, represents all of the regular matter in the universe.
Yet, what if I told you that all of the stars and galaxies and galaxy clusters only comprise 10% of the light sector?
The rest has proved as elusive as the dark sector.
The rest has proved as elusive as the dark sector.
We think it must exist as extremely diffuse gas We think it must exist as extremely diffuse gas in between the galaxies.
Yet, our intense searches miss up to half of it, at least Yet, our intense searches miss up to half of it, at least until now.
until now.
First, a quick refresher on dark matter and dark energy.
And there's plenty more detail in some previous episodes.
Dark matter is believed to be an invisible stuff that interacts only through gravity.
It comprises 80% of the mass of the universe or around 25% It comprises 80% of the mass of the universe or around 25% of its total energy content.
of its total energy content.
Its gravity holds galaxies together and governed to the growth of large-scale structure in our universe throughout cosmic time.
Now where dark matter pools, dark energy pushes.
It's anti-gravitational and causes the expansion of the universe to accelerate.
This energy of the vacuum comprises 70% of the universe's energy content.
The remaining 5% is regular baryonic matter.
The remaining 5% is regular baryonic matter.
By the way, a baryon is a 3-quark particle By the way, a baryon is a 3-quark particle like a proton or a neutron.
But really, baryonic matter refers to atomic matter.
It's the stuff of stars, planets, gas, dust, you, me.
Baryonic matter interacts with light, so we can search for it by scanning the electromagnetic spectrum.
the electromagnetic spectrum.
Except, by doing so, we miss most of it.
Except, by doing so, we miss most of it.
This is the missing baryon problem.
There's a huge discrepancy between the amount of baryonic matter our surveys find and the amount that our theories say should be out there.
And those theories are pretty solid.
For example, in the first several minutes after the Big Bang, hydrogen fused into deuterium and helium.
The proportion of hydrogen that ended up getting fused is very dependent on the density of that hydrogen, so the baryonic mass.
Measuring the relative abundance of helium and deuterium today tells us that there should have been 10 times as much hydrogen to start with than we actually see today in galaxies and clusters.
The second way to calculate the expected baryonic mass is with the cosmic microwave background radiation.
Perhaps, you remember this stuff.
It's the light released at the moment the first atoms formed nearly 400,000 years after the Big Bang.
We still see that light today traveling to us from distant parts.
It carries with it a map of the structure of the cosmos from those early times.
from those early times.
These speckles are fluctuations in density These speckles are fluctuations in density that would later collapse to become the galaxy clusters.
And by analyzing these fluctuations, we can figure out the relative abundance of baryons to dark matter.
See, before the photons of the cosmic background radiation were released, they were trapped in the searing hot plasma of baryonic matter.
The interplay between baryonic and photons resulted in density oscillations.
Much like sound waves rippling outwards from high density regions, these baryonic acoustic oscillations from high density regions, these baryonic acoustic oscillations helped produce a smaller family of speckles helped produce a smaller family of speckles compared to the largest blobs on the CMB map.
Those large blobs are driven by dark matter, which doesn't interact with light, so it can't produce density oscillations.
By analyzing the distribution and speckle sizes in what we call the CMB power spectrum, we can find the relative amount of baryonic versus dark matter.
Again, we calculate that they should be way more baryonic matter than we see in galaxies.
matter than we see in galaxies.
Following the release of the cosmic background radiation, Following the release of the cosmic background radiation, gravity continued to do its work and collapse gravity continued to do its work and collapse these faint fluctuations into gargantuan clusters these faint fluctuations into gargantuan clusters of galaxies.
Supercomputer simulations reveal the shape of this large-scale structure that should result from this gravitational collapse.
It's the cosmic web.
Rivers and sheets of dark matter flow into giant dark matter halos, dragging baryonic matter with them.
In the nexuses between filaments matter is dense enough for galaxies to form.
Now surveys of galaxies confirm that this is what the large-scale structure of the universe is what the large-scale structure of the universe looks like.
looks like.
And yet, when we add up the mass from those galaxies, most of the baryonic matter predicted by a theory is missing.
So where is it?
Well, our best guess is that it's in the form of a very diffuse plasma, atoms in the form of a very diffuse plasma, atoms stripped of their electrons in between the galaxies.
stripped of their electrons in between the galaxies.
Now some of that stuff we can see.
If the plasma is hot enough, then it emits detectable X-rays.
We typically see that stuff inside galaxy clusters where the plasma is relatively dense and is energized by the light of the galaxies themselves.
On the other hand, if the material is cool enough, On the other hand, if the material is cool enough, then nuclei can recapture their electrons then nuclei can recapture their electrons and become a gas instead of a plasma.
This cool gas then absorbs signature wavelengths from light that passes through it.
Absorption features in the light of distant quasars reveal this gas lurking between clusters of galaxies.
However, by looking only at the hottest or the coolest material, we don't find nearly enough of it to account for the missing baryons.
to account for the missing baryons.
It seems that the missing material It seems that the missing material must be in the intermediate temperature range.
It must be hot enough to still be a plasma.
Otherwise, it would produce absorption features.
But it can't be so hot or dense as to emit detectable X-rays.
But it can't be so hot or dense as to emit detectable X-rays.
This tells us that the best hiding place for the missing This tells us that the best hiding place for the missing baryons is the giant filaments that form the cosmic web stretching in between galaxy clusters.
That material would be cooler than the clusters themselves, but should at least be hot enough to form a plasma.
but should at least be hot enough to form a plasma.
See, the vast tidal effects of nearby galaxies See, the vast tidal effects of nearby galaxies create shocks that can heat those baryons to hundreds of thousands or even millions of Kelvin.
At the same time, this stuff is expected to be extremely low-density, only around 10 times that of intergalactic space.
That makes it a more perfect vacuum than anything we've created in a lab or even exists in the Milky Way.
And yet, those filaments are vast, tens of millions of light years long.
And so those solitary baryons could add up to more mass than all of the galaxies in the universe.
So how do we spot this stuff?
So how do we spot this stuff?
Two research groups have figured it out.
Two research groups have figured it out.
The secret is the thermal Sunyaev-Zel'Dovich effect.
We talked about the kinetic SZ effect in our episode We talked about the kinetic SZ effect in our episode on dark flow.
on dark flow.
The thermal SZ is similar.
And again, it makes use of the cosmic microwave background.
As photons from the CMB pass through a giant filament, the hot plasma in the filament grants it a little energy boost.
In fact, the electrons in that plasma scatter CMB photons to higher energies.
So if there's enough of this stuff, then the CMB map should be slightly hotter directly in between galaxies that are connected by filaments.
And it turns out it is hotter.
Two teams, Graaff and collaborators and Tanimura and collaborators, just published the results and collaborators, just published the results of their attempts to look for the Sunyaev-Zel'Dovich Effect.
of their attempts to look for the Sunyaev-Zel'Dovich Effect.
They both used the latest Planck satellite CMB map in the presumed locations of large-scale structure filaments, which they assumed was between pairs of nearby massive galaxies, the type typically found in giant dark matter halos.
found in giant dark matter halos.
Now it wasn't an easy experiment.
Now it wasn't an easy experiment.
The SZ effect is tiny.
And so the researchers needed to add together the results from many, many galaxy pairs.
Graaff et al.
used a million galaxy pairs, while Tanimura et al.
used 260,000.
Both teams report detection of the thermal Sunyaev-Zel'Dovich effect with around 5 sigma significance.
Or to translate, they found the baryons.
These filaments seem to have enough of this hot diffuse plasma to match the amount expected from the models.
We're a very important step closer to accounting for all of the missing baryons.
And this is actually a huge relief.
If our predictions for the relative mass in baryons versus dark matter was so wrong, then it would mean the our understanding of the physics of the Big Bang was seriously off.
So it seems that most of the regular matter in our universe is spread out in the vastness of intergalactic space, still flowing with rivers of dark matter into the galaxy clusters.
As those baryons fall into the dense nexuses of the cosmic web, they'll feed galaxies with material to form new stars.
to form new stars.
In fact, this verifies that the epoch In fact, this verifies that the epoch of star formation in our universe is far from over.
In fact, it's only just beginning.
The stuff of countless future solar systems is still riding the cosmic web, falling in from the darkest reaches of space time.
Support for PBS provided by: