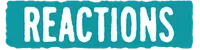
What is an Electron?
Season 8 Episode 1 | 10m 50sVideo has Closed Captions
There is so much more to these “negatively charged particles” than we've thought.
You learned what an electron is in school… or DID YOU? You probably learned that it’s a “negatively charged particle” but there’s *so* much more to these weird, wild little particles than that.
Problems with Closed Captions? Closed Captioning Feedback
Problems with Closed Captions? Closed Captioning Feedback
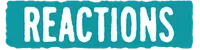
What is an Electron?
Season 8 Episode 1 | 10m 50sVideo has Closed Captions
You learned what an electron is in school… or DID YOU? You probably learned that it’s a “negatively charged particle” but there’s *so* much more to these weird, wild little particles than that.
Problems with Closed Captions? Closed Captioning Feedback
How to Watch Reactions
Reactions is available to stream on pbs.org and the free PBS App, available on iPhone, Apple TV, Android TV, Android smartphones, Amazon Fire TV, Amazon Fire Tablet, Roku, Samsung Smart TV, and Vizio.
Providing Support for PBS.org
Learn Moreabout PBS online sponsorshipIf I asked you to describe this apple, what would you tell me?
You'd probably say something about its size.
It's shape, color.
The fact that it has a thin skin on the outside seeds in the middle, you might tell me about the taste that it grows on trees or that your uncle uses them to make a fine pie.
Shout out to uncle Habib's apple pie.
Even if I had never seen one in my life, I have a pretty good sense of what one is now.
What if I asked you to tell me what an electron is?
How would you describe its size, shape, structure?
What does it look like?
What's it made out of?
How does it interact with the world around it?
How do we use it?
What is it?
Except for how we use it, powering small electronic devices to watch YouTube videos.
For example, we don't have easy answers to any of those questions.
And the more you think about them, the more confusing they seem to become.
Let's start with what seems like the simplest question.
How big is an electron?
Now measuring how big something is in our everyday world is relatively easy, but you cannot measure the size of an electron like this.
They're just too small.
Ironically, to measure the size of something super small, you have to build something really big, a particle accelerator.
Particle accelerators shoot beams of particles at each other at speeds very close to the speed of light, almost 300 million meters per second.
How those particles interact with each other during and after the collision can tell you roughly how big they are.
Here's an analogy.
These are small magnets that I have super glued to the end of sticks, but I want you to think of them as electrons being shot at each other in a particle accelerator.
If I bring together two low energy electrons, at some point they will repel each other and deflect away without touching.
Now, if I increase their energy, the deflections will happen at smaller and smaller distances until eventually I’m bringing them together with enough energy that they'll actually touch each other and bounce off each other.
Ah!
Ah!
At this point, no matter how much extra energy I add, they'll still bounce off each other at exactly the same distance.
And the same sort of thing happens in a particle accelerator with electrons, or at least we think it would happen.
If we were able to accelerate the electrons enough.
Currently we've been able to do some up to about 200,000 times the energy they have just sitting around, but that's not enough to detect any sort of hard core, which is the dense hardball where the guts of the electron would be concentrated.
So all we know for sure about the size of an electron is that it's smaller than 10 to the minus 18 centimeters.
That's about 170,000 times smaller than a helium nucleus.
Some scientists think that if you go down to 10 to the minus 20 centimeters, you'll finally find the metaphorical center of the Tootsie Pop.
Others think that you'd have to get down to 10 of the minus 33 centimeters.
And once you're there, you'd find that electrons don't have a hardcore at all, but are strings.
That's string theory.
And that's a whole other video.
Because we haven't figured out how big electrons are, theoretical chemists and physicists treat them as point-like particles.
In other words, as if they have no size, no diameter as if they have zero volume.
Now electrons do have mass.
one electron weighs about nine times 10 of the minus 31 kilograms.
Now think about that for a second.
Nothing in your everyday behaves like this; has mass, but no volume.
Imagine saying I'm gonna treat this 200 gram apple as if it has zero volume.
That makes no sense.
But with electrons it works.
It doesn't break physics.
It doesn't break chemistry.
It just breaks our brains.
Then there's something called spin half.
Now let's start with the spin part.
First means that electrons behave as though they are little spinning balls of charge.
The half part means that you have to rotate an electron 720 degrees, two full turns to get it back to its original spin state.
Now, given that we don't know the exact size or shape of an electron and treat them as though they have zero volume, it can be hard to get your head around what it means to rotate them 720 degrees.
This video from Lloyd Watts shows a good visual analogy.
This strange looking gear thing is spin one half in that you have to rotate it 720 degrees to get it back to its starting point.
Obviously though, electrons are not super small wooden gears.
One of the defining features of spin one half particles like electrons, protons and neutrons is that they take up space.
And the fact that they do take up space, particularly the electron, determines a lot of everything around us.
Now this is where things get philosophical.
Because I just told you that scientists treat the electron as if it has zero volume, which you'd think means that it takes up zero space, but electrons do take up space and to see how let's look at this hydrogen atom.
It has one proton and one electron.
We can't say exactly where the electron is at any given time, but we can draw shapes around the nucleus that represent regions with a high probability of finding the electron.
These shapes are called orbitals.
So while the electron itself has zero volume, in another sense, it takes up a relatively large chunk of space within an atom.
There's one other important piece to this puzzle.
All particles with spin one half, including electrons, have a fundamental property that says that only one electron can be in an orbital in a given spin state at a given time.
And electrons can either be spin up or spin down.
So each orbital can hold one spin up electron and one spin down electron.
And that is it.
This means there is a fundamental limit on the number of electrons that can fit into a given space in an atom.
Each electron takes up space and pushes other electrons away.
Even though each electron itself has zero volume.
By the way, you probably learned this concept in high school chemistry as the Pauli Exclusion Principle.
It's why you filled atomic orbitals with only two electrons, one spin up and the other spin down.
But it also applies when electrons from two different atoms approach each other.
And that's when the definition of taking up space starts really making sense to show you I'm gonna need a book.
And I just so happen to have one.
Oh I think this one should do it.
When I hit this book, why doesn't my hand go through it?
More specifically, why doesn't the cloud of electrons on the out's surface of my fingers go through the cloud of electrons on the cover of the book.
Now you might think you know the answer to this question and yes, electrons are negatively charged and like charges do repel each other.
So the electrons on the very outermost surface of your hand, repel the electrons on the very outermost surface of the book before my hand even makes contact with the book.
But that's not the only thing happening here to see what's really going on.
Let's assume that my hand and the book are both made of neutral helium atoms.
Now I'm choosing helium because it's one atomic orbital is full of electrons.
It's what your high school chem teacher called happy.
Even though your skin and the book are definitely not made of helium, they're both made of long and complicated molecules.
The vast majority of the atoms in those molecules are happy, meaning they have their full compliment of electrons.
So helium gives a reasonable picture.
Okay.
So each helium atom has two electrons in its one S orbital.
If these two orbitals were to overlap in space, in other words, if my hand and the book were to take up the same space, then we'd have two, two spin up electrons and two spin down electrons in the same orbital in this same space.
And here's the thing.
The universe literally does not allow that to happen.
Why not?
We don't exactly know.
All we know is that if we allow, for example, two spin up electrons to occupy the same orbital, we'd end up with things like negative probabilities affect not following causes and other deeply, deeply weird behavior.
So when the book is taking up this space, my hand cannot also take up this space because identical electrons cannot overlap.
You shall not PASS!
This is so fundamental that it sounds obvious, but let's consider something that is in many ways, the opposite of an electron, a particle of light, a photon.
Photons and electrons can be described by the same fundamental equations.
The difference between them is that while electrons are spin half, photons are spin one.
So instead of needing to turn them two full turns to them back to the same spin state, you only need to turn them one full turn.
Just like literally everything you see around us.
If I turn this apple 360 degrees, it comes back to the same place that it started at.
Now in that sense, photons are not as weird as electrons, but in other ways they're less intuitive.
Look at these two lasers.
They're both shooting out red photons.
Laser on the left is way more intense.
Meaning it's shooting out way more photons than the one on the right, which you can clearly see because it's much brighter.
Now look at the width of the beams.
It's the same.
Identical.
And yet the beam on the left is brighter.
What does that tell you?
And it tells you that say 300 photons can take up the exact same amount of space as 100 photons.
How is that possible?
Well, photons are spin one particles and spin one particles are not subject to the Pauli Exclusion Principle.
Why not?
We have no idea.
The universe is just cool with letting hundreds, thousands, trillions of spin one particles, hang out in the same space.
There's actually no upper limit to the number of photons that can occupy the same space.
We can cram as much light as we want into as small a space as we want.
In fact, with light, the word cram has no meaning since adding additional photons doesn't reduce available space for more photons until you add so many that the system collapses into a black hole.
At that point, you've got bigger problems.
Here's a thought.
Imagine if somehow we were made of photons instead of protons, neutrons and electrons.
Now that statement is fundamentally weird on many different levels, but just roll with it here.
Two humans or five or a thousand could take up the exact same space.
But of course, if we were made of photons, we wouldn't be, well, human.
The fact that spin one half particles like electrons, protons, and neutrons take up space is literally the reason that matter, all stuff, as we know it exists.
If these particles were spin one, they'd have a wispy existence passing through each other like ghosts, no rocks, no floor, no earth, no animals, no plants, no us.
So to get back to where we started, what is an electron?
Well, it's a particle with charge and spin.
It may or may not have any volume, but we treat it as if it has none.
And despite having no volume, it definitely takes up space to the extent that it's one of the fun building blocks of all matter in our universe.
So to summarize an electron is, well, I mean, it's kind of like a, well, if you, if you took a, if you took a tiger and uh, no, that's not right.
Support for PBS provided by: